by Dave Nordling, Secretary, Reaction Research Society
I was recently asked for advice on the installation of a burst disk in a university liquid rocket project. As any pressure relief device is an important subject to consider carefully, I wanted to present a summary of my thoughts to our broader readership.
The Reaction Research Society (RRS) is happy to offer advice, but my first recommendation to any university team would be to talk with your university professors, professional advisers and mentors that are involved with your project. A burst disk is an important component and its function can be critical for safety and preserving your vehicle in any over-pressurization scenario. The subject of your rocket system pressurization, venting and relief devices is extremely important to study well and thoroughly understand before proceeding with any component selection or testing. Your university is the best place to start.
For those who are doing a liquid rocket project outside of a university program, I would also recommend to consult with experts and reputable manufacturers and distributors of pressure relief devices including burst disks.
Burst discs (the spelling “disk” or “disc” is interchangeable) are one simple form of a pressure relief device or valve that is designed to prevent over-pressurization of a pressure vessel and potential catastrophe. Burst disks are also sometimes called “rupture disks” which clearly describe their function.
https://en.wikipedia.org/wiki/Rupture_disc
In liquid rocket system designs, burst disks are often placed not only at the pressurant bottle to protect the higher pressure part of the system, but also at the lower pressure end of the regulator which protects the propellant tanks being pressurized. In the event of pressure regulator failure, the burst disk can protect the propellant tank.
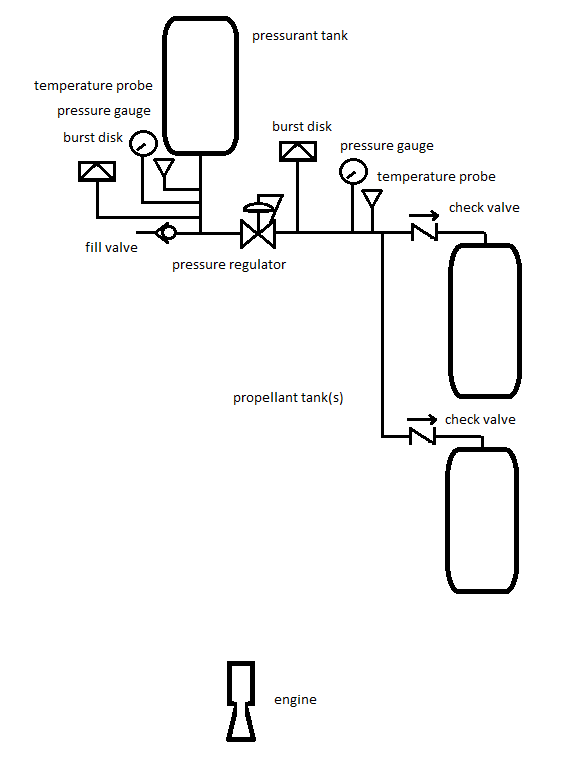
Burst disks are usually in the form of a dead-ended pressure fitting that is adapted to directly connect into the pressure vessel either directly into the pressure vessel volume boundary itself or by a tube connection that is also directly connected into the pressure vessel volume boundary. These fittings have a frangible or breakable membrane that is designed to fail when the pressure reaches a specific design point.
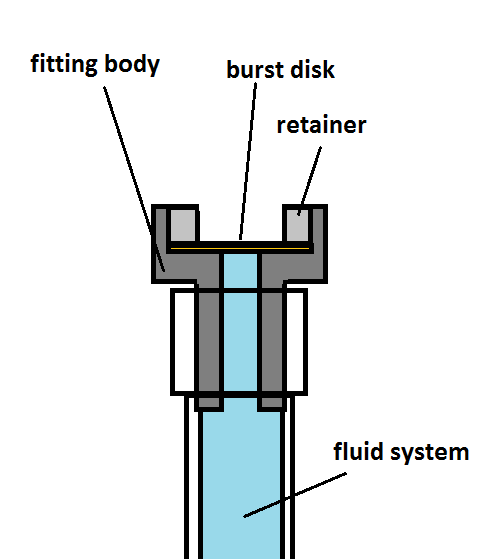
A burst disk is a “one-time use” device and can not be reset after they have “actuated”. As a pressure relief device, the burst disk is often chosen for its compact size and simplicity. They are in common usage in many industries and can fulfill their relief function very well if they are sized and located properly.
They must be securely and directly connected into the volume of the pressure vessel and have no valves or other hardware which would isolate, block, impinge or constrain the relief function in any way. The American Society of Mechanical Engineers (ASME) Boiler and Pressure Vessel (B&PV) code does have some general advice on this subject and this is a good place to start your study.
These devices are simple to understand but fairly complex to size properly. Beyond the design of the burst disk, you must also consider where these devices will physically fit on your vehicle, where are they located and what is the environment doing around your relief device
The burst disk body and membrane can be subject to corrosion or physical damage that could reduce it’s effective bursting pressure. It’s important to consider the material compatibility of all body, seals and membrane materials that are exposed or “wetted” to the gases inside. Also, its important to avoid getting gouges, nicks or marks on the membrane that would form stress concentrations and weaken the membrane. Even when being cautious, don’t leave your burst disk covered when it needs to be ready to perform. Careful handling is good advice at all points in the project.
There are three things to consider when locating and installing a burst disk:
(1) relief (set) pressure, (2) minimum flow rate required, (3) where is your burst disk pointed?
(1) Set pressure of the relief device
Any relief device must be set to actuate (or in the case of a burst disk, to rupture) at a pressure above all of your nominal conditions, but also adequately below any and all failure modes. In some pressure vessel or relief device codes, there are rules of thumb about the set pressure must be a specific percentage (%) above the maximum expected operating pressure (MEOP) or maximum allowable working pressure (MAWP). The thorough examination of all operating conditions and hardware limitations is essential of finding the right set pressure for the relief device.
ASME also has codes for sizing relief valves in process piping, but the rocket industry doesn’t have a particular specification. The aerospace industry does often draft their own specifications and requirements which follow good industrial practices and always include careful design and testing as part of proving the designs to be sufficient.
Another consideration beyond the static pressure in your pressure vessel is the temperature environment of the gases inside. Beyond the fact that higher temperatures from a thermodynamic standpoint create higher pressures, a burst disk relies on the material strength of the membrane and the yield and ultimate strength can weaken under higher temperatures. Some materials (examples are low quality steels) can also become weaker under cold temperatures. Always consider the full range of temperature environments in every application. It’s important to size each burst disk individually and resist the temptation to assume that one device will suit all environments.
There’s a big tolerance on a burst disk set pressure, so be aware of that imprecision. Burst disks are compact but getting a membrane to burst at an exact pressure is not really practical and thus these devices are not very precise. Ask the manufacturer about the expected tolerance on any relief device. It’s also wise to test a few of these devices to measure the actual burst pressure. Make sure you are recording data because failure happens suddenly and you are unlikely to visually see the last pressure reading before burst. If you blink, you can miss the most important data point. Therefore, use a data acquisition system when testing your pressure relief devices.
(2) Minimum flow rate required
Any pressure relief device when activated must be able to drop pressure fast enough to avoid over-pressurizing and failing the pressure vessel. This is a less commonly evaluated situation but its equally important to recognize any scenarios where the transient pressure rise would challenge the relief flow rate needed to keep the pressure below a safe level at all times. Steam pressure systems have this problem and so do cryogenic vessels. Most designers just choose a fitting similar in size to the lines being used, but this isn’t always accurate.
Relief devices are nearly always sized relative to their flow rate afforded. This is sometimes called the “capacity” of the relief valve or burst disk. You’ll need to know your gas and upstream conditions. With this, you’ll need to know the open area when the valve is opened and make this is the smallest restriction in the entire flow path. The open area can be expressed as either the discharge area (Cd A) or the valve coefficient “Cv” value. With each device in each specific location, you must select a burst disk capable of venting enough flow to cover the whole range of expected conditions. This is crucial to finding the right burst disk or relief valve. A device that does not have a large enough capacity will not protect your fluid system.
Another consideration for your relief device is if you have any flow path that is smaller than the area of your relief device. One example of poor design is having your pressure relief device located at the end of a long skinny tube. Even if the open area of the tubing is larger than the pressure relief valve opening, the length of the line can accumulate enough flow friction in the tubing that can unintentionally add up enough pressure drop to pose a significant restriction to your relief flow. This is to say nothing of someone accidentally denting or kinking the tubing which would create a severe blockage of the relief flow. It’s always smart to have your pressure relief device very closely coupled to the pressure vessel volume that you are protecting. This means keeping the distance as short as possible. Always know all of your flow path areas and line lengths!
Another classic mistake in fluid system design is putting a valve or any other restriction device in-between the pressure boundary volume and the pressure relief device that is protecting it. Careful consideration of all valve placements and their positions in all operating modes and under all possible operating scenarios. Put simply: “Do NOT EVER create a situation where the pressure relief device can be isolated or impeded in its operation at any time for any reason, even temporarily. Some piping codes absolutely forbid this. Careful peer-review of your pressure and instrument diagrams (P&ID’s) must look for this situation and avoid it. More than reviewing the paper schematics, one should physically trace all flow paths to be sure the builder hasn’t made such a mistake. The physical hardware must always match the P&ID.
(3) Watch where your burst disk is pointed!
When your burst disk goes off, any foreign object debris (FOD) near the discharging outlet can be thrown out at high speed causing injury or damage to nearby hardware and structures. Even without particulates or FOD, the impinging high-speed sonic jet of gas is very dangerous. No one should be standing near a fluid system while any part of it is pressurized anyway, but you should always consider what might happen when your burst disk goes off. You won’t always know when the device will go off. Be prepared at all times.
Make sure all hardware is also secure enough to take the sudden thrust from the burst disk relieving itself. This can be a sudden and powerful force that breaks hardware or knocks things over. The rocket thrust equation also applies in this case. To calculate this thrust value, you do this in two parts: (1) You consider the choked flow pressure differential multiplied by the discharge area and (2) add in the product of the mass flow rate of the gas escaping multiplied by the sonic velocity of the upstream gas conditions.
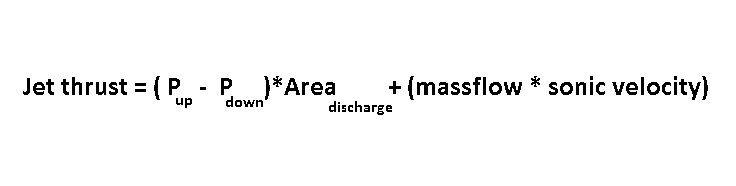
As a design note, for nearly all gases, if the upstream pressure is more than double that of the downstream pressure, the flow velocity through any flow path restriction(s) or “orifice area” is sonic or at the speed of sound as computed by the upstream gas pressure and temperature conditions. This is called “choked” flow.
One potential fix to the jet thrust problem out of relief device is to divert and diffuse the discharging outlet flow in opposing or evenly distributed directions as long as the combined discharge flow areas are sufficiently large and balanced.
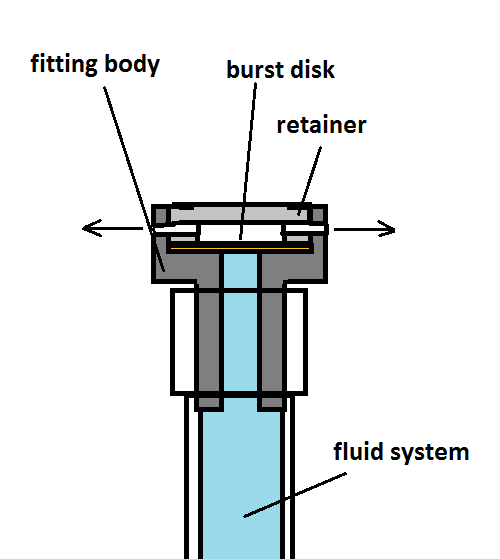
Another consideration to be made with a burst disk or pressure relief device is to consider the downstream environment where your burst disk is discharging.
Is the relieving gas or gas mixture going to create a flammable or toxic environment? If so, you need to consider how and where you are diverting the hazardous gases being relieved. Some burst disk fittings have threaded ends on both ends which allows the discharging flow to be routed to a safe location, if this is a necessary feature.

Are you creating a dangerous environment (reduced oxygen) within a confined space? The subject of confined space safety is very important and worthy of a separate article in itself. Most testing will be done outdoors and in a very well ventilated environment, but the rocket business is full of horror stories of people who have become injured or asphyxiated simply from improper consideration of confined space safety.
A less often considered scenario is whether the space where the burst disk or relief valve is discharging into is fully open to the environment or not. It is possible to overly restrict or “back up” a burst disk or relief valve if the interstage volume in your rocket isn’t very large or isn’t adequately vented to the outside. Sometimes your discharge space simply isn’t big enough. It is very important to know your vehicle hardware geometry very well, measure your volumes and consider all flow areas out of all assemblies.
Find a reputable burst disk manufacturer and distributor
There are a few reputable manufacturers of burst disks. Fike is one that comes to mind, but they tend to be for very large piping sizes used in facility plants. Fike has been providing reliable products for many years to many industries including oil/gas and the aerospace industry. Swagelok has access to a lot of fluid component manufacturers which may be more suitable.
Zook is another manufacturer of burst disk fittings. These in-line devices come as a holder fitting and replaceable disk. The screw-type fittings are two-piece assemblies and have standard pipe thread ends. The disks come in a range of nominal set pressures.

zookdisk.com
There are certainly other manufacturers and all of them should be able to provide you with good advice or transfer you to a distributor company to help you with selecting an appropriate device. Before you call or email, you must have already taken the time to understand your pressure environment, capacity and design requirements first. A good component distributor is one that is willing to work with you to find the right part for your application and educate you in making the best choice. Literature is easy to find online and always consider more than one manufacturer to get a good price.
A few last words of caution
Burst disk devices can be manufactured from scratch and other amateur rocketry hobbyists have attempted to do so. This is not a good idea. There are a lot of considerations to make in building a reliable burst disk from scratch not to mention the time and materials to adequately prove the design. To make a burst disk from scratch would become every bit as expensive as simply going to a reputable manufacturer and using their product.
As much as your group may want to save money, pressure relief devices are a critical part of your fluid system to which lives may be at stake. Don’t be cheap. Find a quality manufacturer, select the right product and test them. Ebay is not the place to find quality products.
If anyone has anything to add to this subject, please contact the RRS secretary or the RRS director of research.
secretary@rrs.org
research@rrs.org